What is Metallic Bonding?
Metallic bonding is a type of chemical bonding that occurs uniquely in metal elements, characterised by a shared pool of electrons. This section will break down the fundamental aspects of metallic bonding.
Key Characteristics of Metallic Bonds
Electrostatic Attraction: The core of metallic bonding lies in the electrostatic forces between positively charged metal ions (cations) and negatively charged electrons.
Delocalised Electrons: Electrons in metallic bonds are not fixed in place between two atoms. Instead, they move freely throughout the metal lattice, contributing to the metal's conductivity and other properties.
Giant Metallic Lattice: The structure of metals can be visualised as a lattice - a repeating, orderly arrangement of atoms that extends throughout the material.
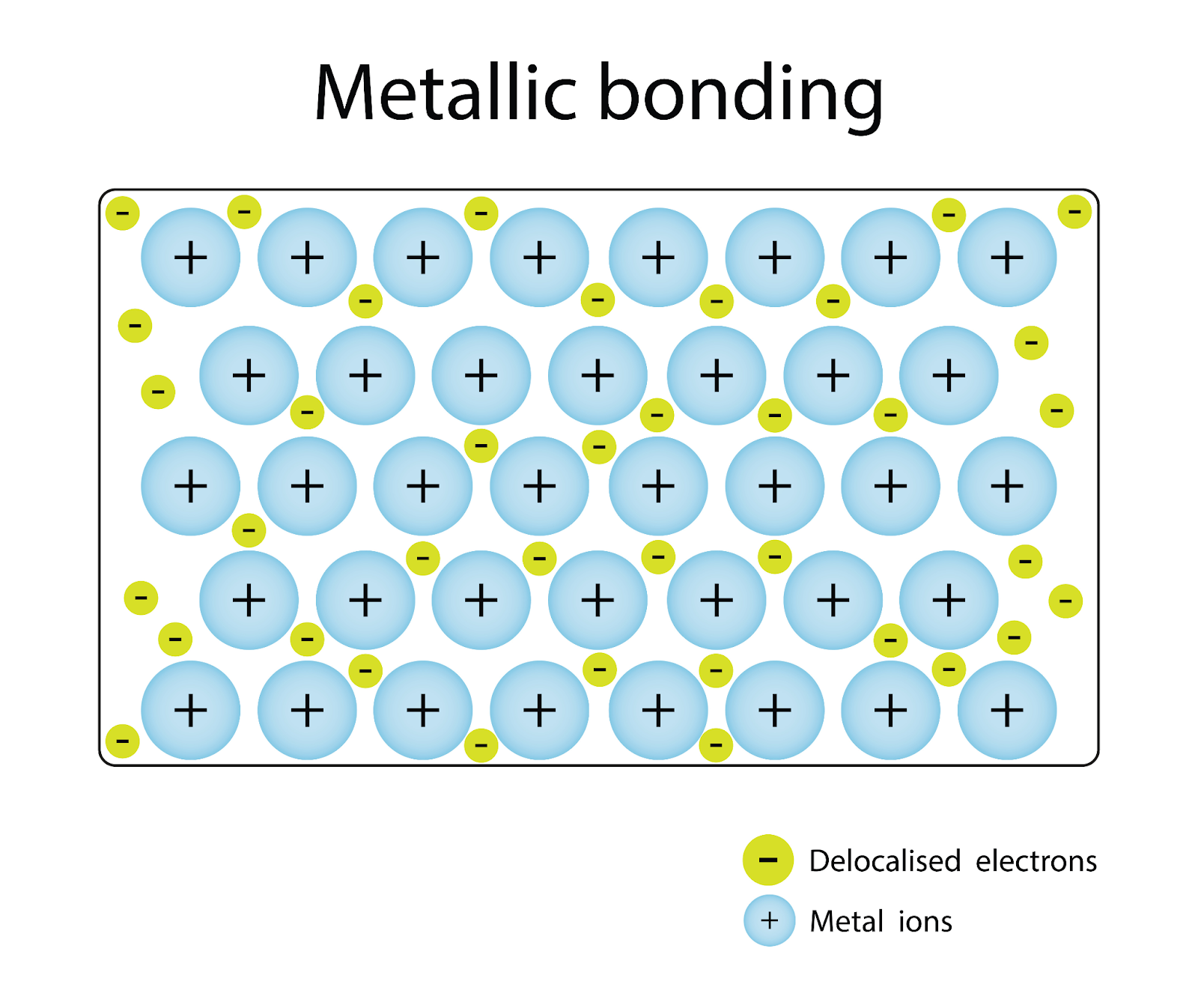
Image courtesy of Watthana Tirahimonch
Formation and Nature of Metallic Bonds
Process of Bond Formation
Loss of Valence Electrons: Metal atoms, characteristically having few electrons in their outer shell, tend to lose these electrons easily.
Formation of Positive Metal Ions: As a result, metal atoms become positively charged ions, while their lost electrons become delocalised.
Attraction Between Ions and Electrons: The positive metal ions are held together by their collective attraction to the sea of delocalised electrons.
Detailed Structure of the Metallic Lattice
Close Packing: Metal atoms are packed closely together in a fixed arrangement, which can be either body-centred cubic, face-centred cubic, or hexagonal close-packed.
Uniform Distribution of Electrons: The delocalised electrons are evenly spread across the lattice, creating a stable structure.
Properties of Metals Arising from Metallic Bonds
Electrical Conductivity
Free Electrons: The freely moving electrons can carry electrical charge through the metal, making metals good conductors of electricity.
Influence of Temperature: The conductivity of metals typically decreases with increasing temperature due to increased atomic vibrations.
Thermal Conductivity
Transfer of Kinetic Energy: The same free electrons that conduct electricity can also transfer kinetic energy, contributing to the high thermal conductivity of metals.
Malleability and Ductility
Layered Atomic Arrangement: The ability of metal atoms to slide over each other without breaking the metallic bond makes metals malleable (able to be hammered or pressed permanently out of shape without breaking) and ductile (able to be drawn into a wire).
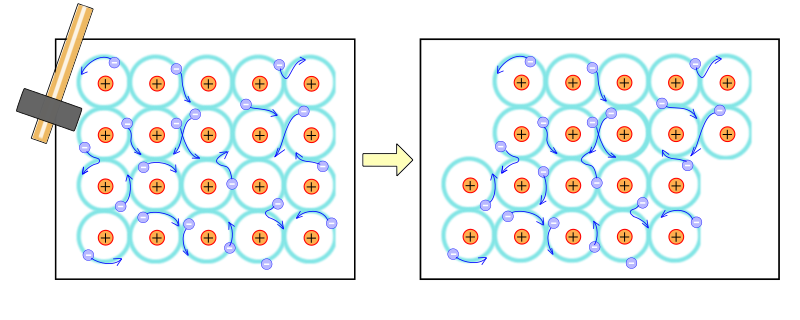
Image courtesy of すじにくシチュー
High Melting and Boiling Points
Strong Bonding Forces: The strength of the bond between the ions and the electron sea results in metals typically having high melting and boiling points.
Lustrous Appearance
Interaction with Light: The delocalised electrons can absorb and re-emit photons of light, giving metals their characteristic shine.
Comparative Analysis of Metallic Bonding
Metallic vs Ionic Bonding
Ionic Bonding: Involves a complete transfer of electrons from one atom to another, resulting in the formation of charged ions.
Comparison: Metallic bonding, unlike ionic bonding, does not form discrete molecules but instead forms a continuous structure of ions and electrons.
Metallic vs Covalent Bonding
Covalent Bonding: Involves the sharing of electrons between two specific atoms, forming a molecule.
Comparison: In metallic bonds, electrons are not shared between fixed atoms but are free to move throughout the entire metal structure.
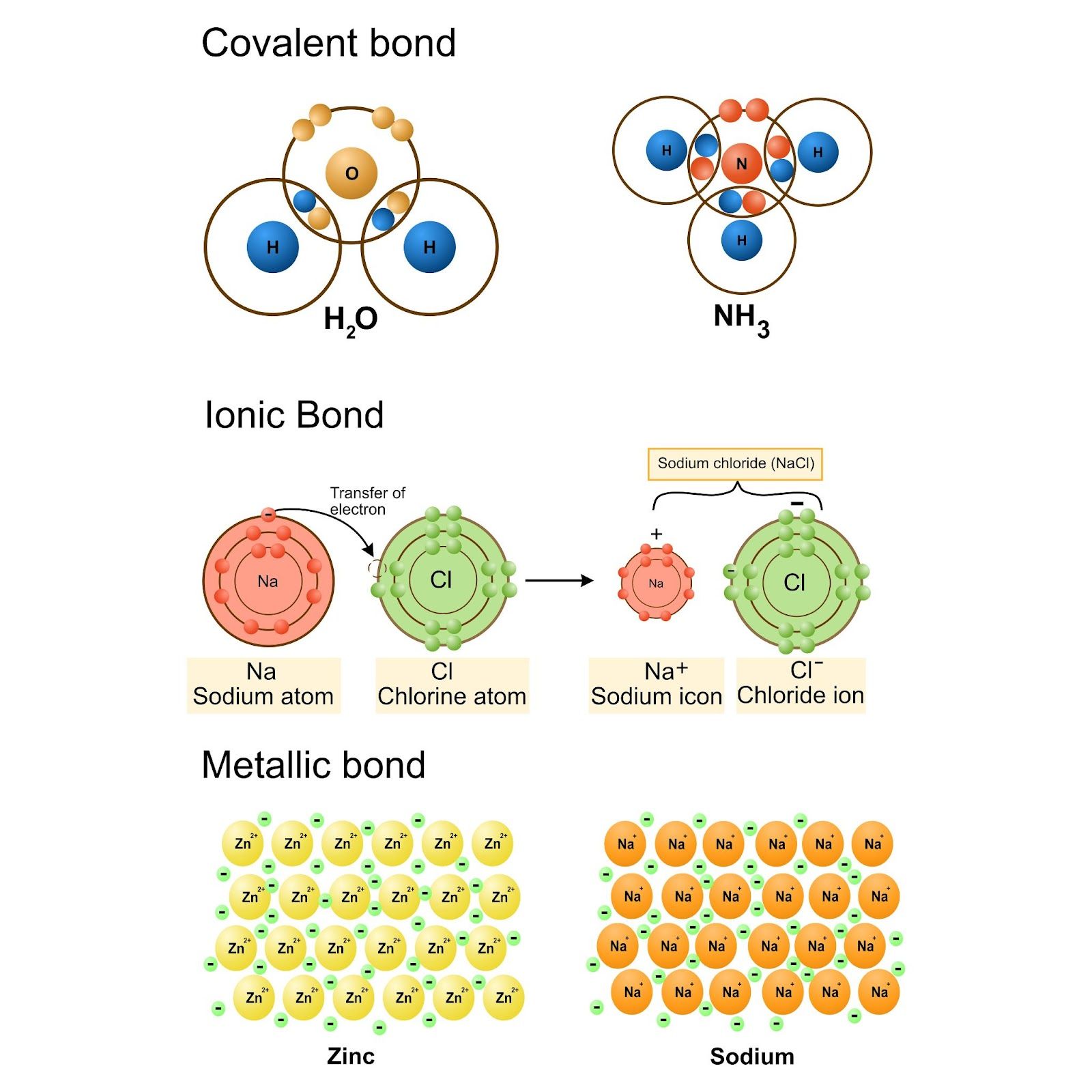
Image courtesy of anchalee thaweeboon
Real-World Examples and Applications
Everyday Metals
Iron (Fe): Strong metallic bonds contribute to its use in construction and manufacturing.
Gold (Au): Its malleability and ductility, due to metallic bonding, make it ideal for jewellery and electronics.
Alloys
Stainless Steel: An alloy of iron, chromium, and nickel, exhibits enhanced properties like corrosion resistance due to the nature of metallic bonding in its constituent metals.
Bronze: An alloy of copper and tin, showcases how metallic bonding contributes to improved hardness and melting point compared to its component metals.
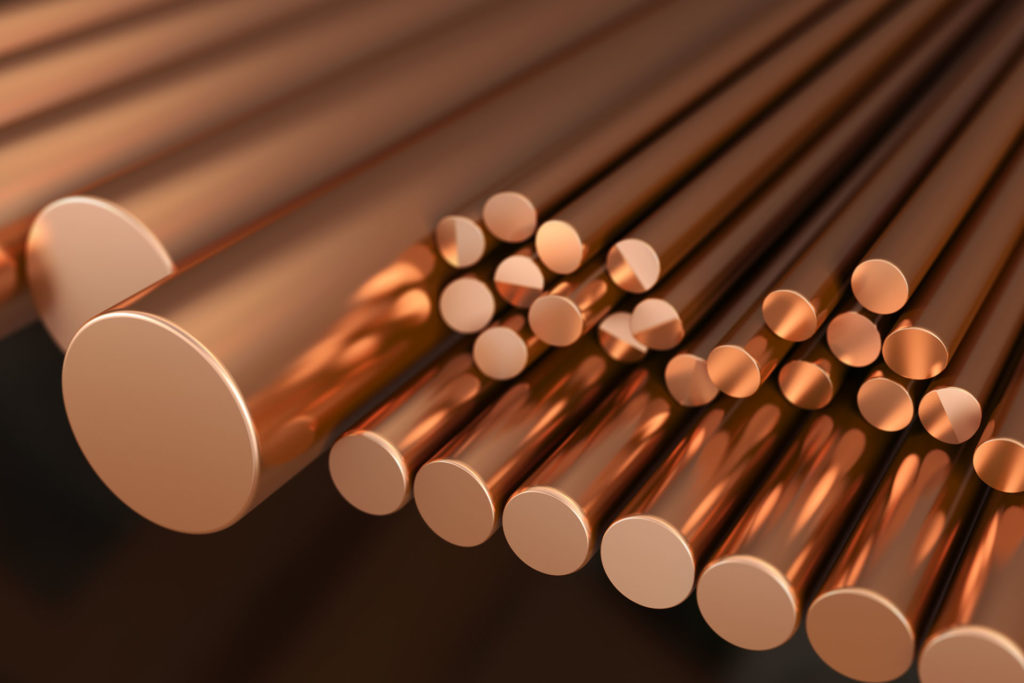
Image courtesy of Fine Metal
Significance in Advanced Technologies
Semiconductors: Some metals, when combined with non-metals, can exhibit semiconductor properties, pivotal in electronics.
Superconductors: Certain metals and alloys, at very low temperatures, exhibit superconductivity, a property underpinned by the nature of metallic bonding.
In conclusion, metallic bonding is a cornerstone concept in chemistry, especially in understanding the behaviour and applications of metals. This type of bonding is distinguished by its electrostatic attraction between positive metal ions and a sea of delocalised electrons, which imparts metals with their characteristic properties like conductivity, malleability, and high melting points. The study of metallic bonding is not only crucial for academic purposes but also for its practical applications in various fields, including materials science, engineering, and technology.
FAQ
Yes, metallic bonding does occur in alloys and plays a significant role in their properties. Alloys are mixtures of two or more elements, usually metals, where the main component is a metal. In alloys, metallic bonding occurs between the different types of metal atoms present. The introduction of different atoms into a metal's lattice can strengthen the overall metallic bonding. This is because the different sizes and properties of the alloying elements can disrupt the uniformity of the metal lattice, making it harder for layers of atoms to slide past each other, as seen in pure metals. This disruption often leads to enhanced strength, hardness, and resistance to corrosion, compared to the pure metals. For example, steel, an alloy of iron and carbon, is much stronger and more durable than pure iron due to the presence of carbon, which affects the metallic bonding and lattice structure of iron.
Most metals are solid at room temperature due to the strong electrostatic forces of attraction in metallic bonds, which hold the metal ions in a fixed, close-packed lattice structure. These electrostatic forces between the positively charged metal ions and the sea of delocalised electrons are strong enough to keep the atoms in a rigid arrangement. Despite the electrons being delocalised and mobile, the ions themselves are not, thus maintaining the solid state. The energy at room temperature is not sufficient to overcome these strong bonds and allow the atoms to move past each other into a liquid form. Therefore, the strength and nature of metallic bonds are key in maintaining the solid state of most metals under normal conditions. Only metals with relatively weaker metallic bonds, like mercury, exist as liquids at room temperature because their bonding structure requires less thermal energy to overcome.
The 'sea of electrons' in metallic bonding significantly influences a metal's reaction to magnetic fields. In metals, the delocalised electrons, which are free to move throughout the metal lattice, are also able to align their spins in response to an external magnetic field. This alignment of electron spins contributes to the metal's magnetic properties. In ferromagnetic materials, such as iron, nickel, and cobalt, the domains of aligned spins become permanently aligned in the presence of a magnetic field, making the material itself magnetic. In contrast, paramagnetic materials, which also have a sea of delocalised electrons, only exhibit magnetic properties in the presence of an external magnetic field and lose these properties once the field is removed. The behavior of these electrons in the presence of magnetic fields underpins the magnetic properties of different metals and is a direct consequence of the nature of metallic bonding.
Metals with strong metallic bonds tend to have higher melting points due to the greater amount of energy required to overcome these bonds. The strength of a metallic bond is largely determined by two factors: the number of delocalised electrons and the charge and size of the metal cations. Metals with more delocalised electrons (typically those with more valence electrons to lose) have stronger bonds as there is a greater electrostatic attraction between these electrons and the positive ions. Additionally, smaller and more highly charged ions create a stronger attraction to the electron sea. This strong attraction in metals with strong metallic bonds means that more thermal energy is needed to provide the ions enough kinetic energy to overcome these attractions and transition from a solid to a liquid state. Hence, metals like tungsten, which have strong metallic bonds, have high melting points.
The concept of metallic bonding explains the ductility of metals through the ability of metal atoms to move past each other without breaking the bond. Ductility refers to a material's ability to be stretched into a wire. In metals, the delocalised electrons in the metallic bond create a fluid-like sea around fixed cations. When a metal is stretched, these cations can slide over each other while still being immersed in the sea of delocalised electrons. This movement does not disrupt the metallic bond because the electrons are not fixed to any particular atom, allowing the metal to stretch without the bond breaking. The ability to maintain cohesive strength while the atoms are rearranged under tensile stress is what imparts metals with their ductile property. This characteristic is particularly useful in applications where metals must be drawn into wires or threads, such as in electrical wiring or cable manufacturing.
Practice Questions
The high electrical conductivity of metals is a direct result of the structure of metallic bonds. In metallic bonding, the outer electrons of the metal atoms are delocalised, meaning they are not bound to any specific atom but are free to move throughout the metal lattice. This 'sea' of free-moving electrons allows electric current, which is essentially a flow of electrons, to pass through the metal with ease. When a voltage is applied, these delocalised electrons can move freely towards the positive terminal, thus carrying the electric current across the metal. The densely packed lattice of positively charged metal ions also facilitates the movement of these electrons, contributing further to the metal's ability to conduct electricity efficiently.
Metallic bonding is responsible for the malleability of metals due to the unique arrangement of atoms and electrons within the metal lattice. In metals, atoms are arranged in layers, held together by a sea of delocalised electrons. This structure allows the layers of atoms to slide over each other when force is applied, without breaking the metallic bond. As the bonds are not directional and the delocalised electrons can move freely to accommodate the movement of atoms, the metal can be hammered or bent into different shapes without fracturing. This ability to deform without breaking is what defines the malleability of metals and is a direct result of the nature of metallic bonds.